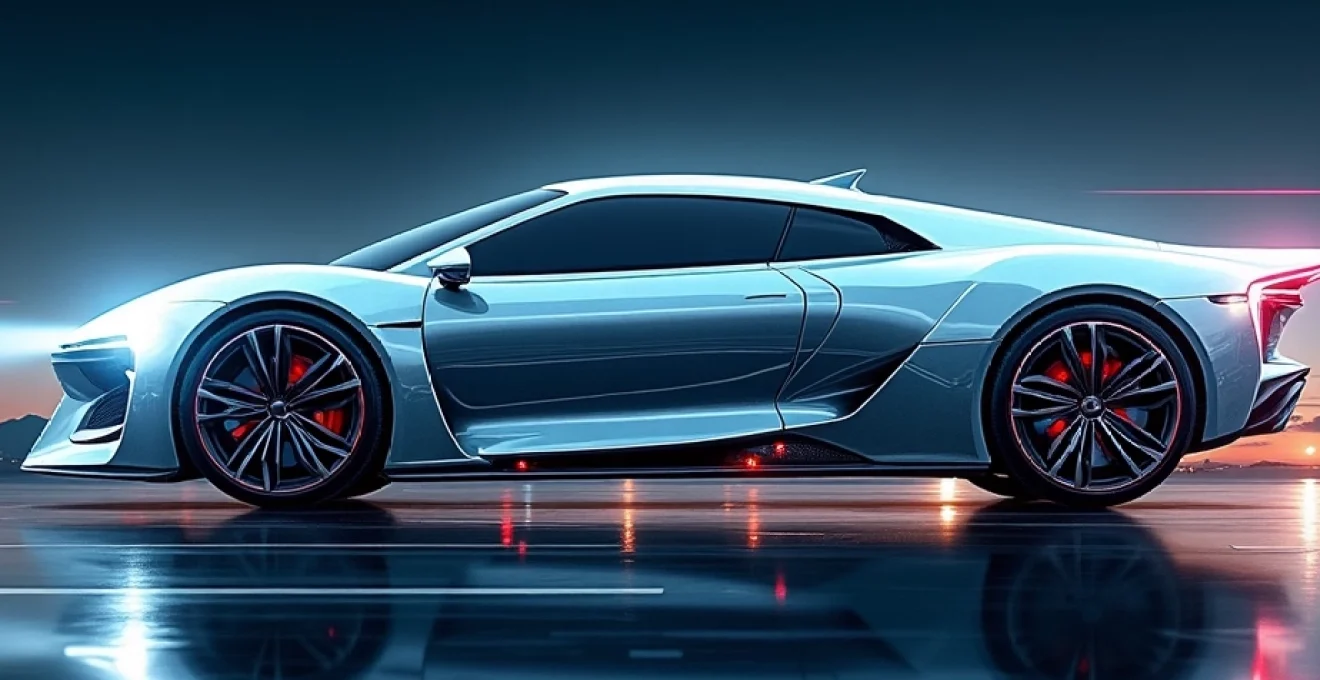
Hybrid vehicles represent a significant leap forward in automotive technology, blending the best of internal combustion engines with electric powertrains. These innovative machines offer improved fuel efficiency, reduced emissions, and enhanced performance, making them an increasingly popular choice for environmentally conscious drivers. As the automotive industry continues to evolve, hybrid technology stands at the forefront of the transition towards more sustainable transportation solutions.
Evolution of hybrid powertrain technologies
The journey of hybrid vehicles began in the late 20th century, with early experiments paving the way for the sophisticated systems we see today. Initially, hybrid technology focused primarily on improving fuel economy. However, as the technology matured, engineers discovered ways to leverage electric motors to enhance performance as well.
One of the most significant milestones in hybrid technology was the introduction of regenerative braking. This innovative feature allows hybrid vehicles to capture energy typically lost during deceleration and convert it into electricity, which is then stored in the battery for later use. This breakthrough dramatically improved overall efficiency and extended the electric-only range of hybrid vehicles.
Another crucial development was the refinement of power management systems. Early hybrids often struggled with seamless transitions between electric and gasoline power, resulting in a less-than-smooth driving experience. Modern hybrid vehicles, however, utilize advanced algorithms and sophisticated hardware to ensure a virtually imperceptible switch between power sources, providing a driving experience on par with or superior to conventional vehicles.
Parallel vs. series hybrid architectures
Hybrid vehicles can be broadly categorized into two main architectures: parallel and series. Each design offers unique advantages and is suited to different driving scenarios. Understanding these architectures is crucial for appreciating the diverse range of hybrid vehicles available in the market today.
Toyota prius: pioneering parallel hybrid system
The Toyota Prius stands as an icon of parallel hybrid technology. In a parallel hybrid system, both the electric motor and the internal combustion engine can directly power the wheels. This design allows for efficient power delivery in various driving conditions. The Prius utilizes a sophisticated power split device, which enables the vehicle to seamlessly transition between electric-only, gasoline-only, and combined power modes.
One of the key advantages of the parallel hybrid system is its versatility. At low speeds or in stop-and-go traffic, the Prius can operate solely on electric power, significantly reducing fuel consumption and emissions in urban environments. When more power is needed, such as during highway driving or rapid acceleration, the gasoline engine seamlessly engages to provide additional thrust.
Chevrolet volt: extended-range series hybrid
In contrast to the parallel system, the Chevrolet Volt employs a series hybrid architecture, also known as an extended-range electric vehicle (EREV). In this configuration, the gasoline engine does not directly drive the wheels. Instead, it acts primarily as a generator to produce electricity, which then powers the electric motor or charges the battery.
The Volt's design allows for a significant all-electric range, typically around 50 miles, before the gasoline engine needs to engage. This makes it an excellent choice for drivers with short to medium commutes who can rely primarily on electric power for daily driving. The gasoline engine provides peace of mind for longer trips, eliminating the range anxiety often associated with pure electric vehicles.
Honda i-MMD: intelligent multi-mode drive system
Honda's Intelligent Multi-Mode Drive (i-MMD) system represents a hybrid approach that combines elements of both parallel and series architectures. This innovative system allows the vehicle to automatically select the most efficient power source based on driving conditions.
In city driving, the i-MMD system primarily operates as a series hybrid, with the electric motor driving the wheels and the gasoline engine serving as a generator. During highway cruising, the system can switch to a parallel configuration, allowing the more efficient gasoline engine to directly power the wheels. This flexibility results in excellent fuel economy across a wide range of driving scenarios.
BMW i3 REx: range extender hybrid concept
The BMW i3 with Range Extender (REx) showcases yet another variation on hybrid technology. While primarily designed as an electric vehicle, the i3 REx includes a small gasoline engine that serves as a generator to extend the vehicle's range. This design is particularly useful for drivers who primarily use their vehicle for short trips but occasionally need to travel longer distances.
The i3 REx operates as a pure electric vehicle until the battery charge drops to a certain level. At this point, the small gasoline engine kicks in to generate electricity, extending the vehicle's range. This approach allows for a larger battery and more extensive electric-only operation compared to traditional hybrids, while still providing the flexibility of a gasoline engine for longer journeys.
Energy management systems in hybrid vehicles
The heart of any hybrid vehicle lies in its energy management system. These sophisticated systems are responsible for optimizing the use of both electric and gasoline power, ensuring maximum efficiency and performance. Energy management in hybrid vehicles involves a complex interplay of various technologies and components, each playing a crucial role in the vehicle's overall operation.
Regenerative braking technology
Regenerative braking is a cornerstone of hybrid vehicle efficiency. This technology captures kinetic energy during deceleration or braking, which would otherwise be lost as heat, and converts it into electrical energy. The recaptured energy is then stored in the vehicle's battery for later use.
The effectiveness of regenerative braking can vary significantly between different hybrid models. Some advanced systems can recover up to 70% of the vehicle's kinetic energy during braking, substantially contributing to overall efficiency. This technology is particularly beneficial in urban driving scenarios with frequent stops and starts.
Power split devices: planetary gear sets
Many hybrid vehicles, particularly those with parallel architectures, utilize power split devices based on planetary gear sets. These ingenious mechanical systems allow for the seamless blending of power from the gasoline engine and electric motor.
A typical power split device consists of a sun gear, planet gears, and a ring gear. By controlling the rotation of these components, the system can efficiently distribute power between the engine, motor, and wheels. This arrangement allows for continuous variable gear ratios, optimizing efficiency across a wide range of driving conditions.
Battery management systems: lithium-ion vs. nickel-metal hydride
The battery is a critical component in any hybrid vehicle, and its management system plays a vital role in ensuring optimal performance and longevity. Two primary battery technologies dominate the hybrid market: lithium-ion and nickel-metal hydride.
Lithium-ion batteries offer higher energy density, allowing for more compact and lightweight designs. They also have a longer lifespan and can charge and discharge more rapidly. However, they are more expensive and require more sophisticated management systems to prevent overheating.
Nickel-metal hydride batteries, while less energy-dense, are generally more robust and cost-effective. They have a proven track record in hybrid applications and can withstand a higher number of charge-discharge cycles. However, they are heavier and less efficient in extreme temperatures compared to lithium-ion batteries.
Hybrid-specific CVT and e-CVT transmissions
Continuously Variable Transmissions (CVTs) and their electric counterparts (e-CVTs) are often employed in hybrid vehicles to maximize efficiency. These transmissions allow the engine to operate at its most efficient RPM range regardless of vehicle speed.
E-CVTs, in particular, are well-suited for hybrid applications. They use electric motors instead of traditional pulleys and belts, providing smoother operation and better integration with the hybrid powertrain. This technology allows for seamless power delivery and contributes significantly to the overall efficiency of the hybrid system.
Performance enhancements through hybridization
While improved fuel efficiency is often the primary focus of hybrid technology, these systems also offer significant performance benefits. The integration of electric motors with traditional gasoline engines can result in enhanced acceleration, improved torque delivery, and even better handling in some cases.
Torque fill: electric motor acceleration boost
One of the most notable performance advantages of hybrid systems is the concept of "torque fill." Electric motors can deliver maximum torque instantly, unlike internal combustion engines that need to rev up to reach peak torque. In hybrid vehicles, the electric motor can provide an immediate boost of torque during acceleration, filling in the lag typically experienced with gasoline engines.
This torque fill effect results in quicker off-the-line acceleration and more responsive performance in everyday driving situations. It's particularly noticeable in stop-and-go traffic or when merging onto highways, where that instant burst of torque can make a significant difference in vehicle dynamics.
Mclaren P1: hybrid hypercar technology
The McLaren P1 serves as a prime example of how hybrid technology can be leveraged for extreme performance. This hypercar combines a twin-turbocharged V8 engine with a powerful electric motor, resulting in a combined output of nearly 1000 horsepower.
In the P1, the electric motor not only provides additional power but also helps to eliminate turbo lag. By filling in the power delivery gaps typically associated with turbocharged engines, the hybrid system ensures instantaneous response and seamless acceleration throughout the rev range. This application of hybrid technology demonstrates its potential not just for efficiency, but for enhancing performance at the highest levels of automotive engineering.
Formula 1 KERS and MGU-K systems
The world of Formula 1 racing has also embraced hybrid technology, further proving its performance potential. The Kinetic Energy Recovery System (KERS) and its successor, the Motor Generator Unit - Kinetic (MGU-K), capture energy during braking and deploy it for short bursts of additional power.
These systems not only improve lap times but also add an extra layer of strategy to racing. Drivers must carefully manage their energy deployment, deciding when to use the extra boost for overtaking or defending their position. The success of hybrid systems in the high-pressure environment of F1 has accelerated the development and acceptance of this technology in consumer vehicles.
Plug-in hybrid electric vehicles (PHEVs)
Plug-in Hybrid Electric Vehicles (PHEVs) represent the next evolution in hybrid technology, bridging the gap between traditional hybrids and fully electric vehicles. PHEVs feature larger battery packs that can be charged from external power sources, allowing for extended electric-only driving ranges.
The key advantage of PHEVs is their flexibility. For short to medium-length trips, they can operate as pure electric vehicles, producing zero emissions. When the battery charge is depleted or for longer journeys, the gasoline engine takes over, eliminating the range anxiety associated with pure electric vehicles.
Many PHEVs offer electric-only ranges of 30-50 miles, which is sufficient for most daily commutes. This capability allows owners to complete their regular driving routines using only electric power, reserving the gasoline engine for longer trips or unexpected detours.
The environmental impact of PHEVs can be significant, especially if the electricity used for charging comes from renewable sources. However, the full benefits of PHEVs are realized when owners have access to regular charging, either at home or at work. Without frequent charging, PHEVs may operate primarily on their gasoline engines, negating much of their potential efficiency gains.
Future trends: mild hybrids and 48V systems
As the automotive industry continues to push for greater efficiency and lower emissions, mild hybrid systems and 48-volt electrical architectures are gaining prominence. These technologies offer many of the benefits of full hybrid systems but at a lower cost and with less complexity.
Mild hybrid systems typically use a small electric motor-generator in place of the traditional alternator. This motor-generator can provide a power boost during acceleration, enable more efficient start-stop systems, and recover energy through regenerative braking. While mild hybrids can't operate on electric power alone, they can significantly improve fuel efficiency, especially in urban driving conditions.
The shift to 48-volt electrical systems is enabling more powerful and efficient mild hybrid setups. Compared to traditional 12-volt systems, 48-volt architectures can handle higher power demands, supporting not only hybrid functions but also other power-hungry features like active suspension systems and electric turbochargers.
Many automakers are adopting these technologies across their model ranges, viewing them as a cost-effective way to meet increasingly stringent fuel economy and emissions standards. As the technology matures and production scales up, we can expect to see mild hybrid systems become ubiquitous in the automotive market, providing a stepping stone towards more extensive electrification.
The future of hybrid technology looks promising, with continued advancements in battery technology, power electronics, and energy management systems. As these technologies evolve, we can anticipate even greater improvements in efficiency, performance, and affordability, further accelerating the adoption of hybrid vehicles worldwide.